The 'Shroom:Issue LXIX/A Genetic Analysis of the Koopas
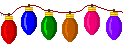
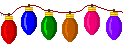
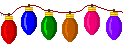
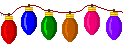
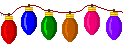
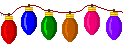
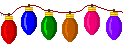
There are few topics in the Mario series that are as polarizing as Bowser's parentage. Originally he had seven Koopaling children, featured in all sorts of games, cartoons and comics, but then they started to appear less and less, and their relationship to Bowser didn't really come up anymore. Many of us were saddened by this, while others were happy to see the Koopalings replaced by more varied world bosses. Then Bowser Jr. came along and really made things interesting: some thought of him as an unimaginative Baby Bowser clone and resented him for "replacing" the original Koopalings, while others accepted him as the eighth Koopaling, and still others reserved their love for Junior alone and jumped on the opportunity to forget Bowser's original kids. Then, when the seven Koopalings came back, feelings were mixed: some were overjoyed, others bemoaned the new designs, and of course, the haters still hated. One thing that didn't change was the ambiguity about how the seven Koopalings fit into the Koopa family - that is, until someone asked Shigeru Miyamoto about it. His answer? Nintendo's "current story" is that Junior is Bowser's only child.
Fond childhood memories were destroyed upon hearing the revelation, while others vowed to keep them related in their headcanons, and some just shrugged. The faction that had always favoured Junior over the Koopalings, on the other hand, were quite happy at this development, and while most played nice about it, there are always a few in every group who just gotta say "I told you so". Now, there are many valid reasons to dislike the Koopalings, and we're all entitled to an opinion, however one of the main arguments that's brought up as to why the Koopalings were never suitable as Bowser's offspring was that they look too different, and that's just not true. I've seen many fans trying to explain their varied by looks by saying they were adopted or had different mothers or whatever, but it is conceivable that they were all produced by Bowser and a single mother, and genetics can show us why.
Yes, real world genetics can prove that they could be related, and because I'm as much of a science nerd as a Mario fan, I'm going to explain how this is so. Obviously none of it is canon, especially in light of Miyamoto's little bombshell, but Nintendo saying they aren't related doesn't mean that from an in-universe perspective, they couldn't be related, and after reading this, hopefully you'll understand why it's misguided to say "they could never have been a family anyway". And who knows, maybe you'll learn a bit about genetics too.
Step 1: Simplify, Simplify, Simplify!
If we were going to go through the genetics for every trait seen in the Koopalings, we'd be here all day, so I'm limiting our scope to the different eye, hair, head and shell colours. For the sake of argument, I'll be including both the seven Koopalings' original designs and their new looks introduced in New Super Mario Bros. Wii.
The main benefit of looking at colours is that they're what's known as discrete traits, which means either you've got 'em or you don't (such as eye colour), whereas most qualities (like height) are continuous traits, and have (in theory) an infinite amount of potential values, rather than a few specific groupings. Continuous traits are controlled by many genes, hence there is a much finer degree of variation in something like height compared to blood type, and while qualitative traits are usually controlled by more than one gene as well, to keep things simple, we're just gonna say there's one gene per trait as far as we're concerned. By default, that means we can't analyze continuous traits like the Koopalings' heights or builds, and while their various powers are discrete traits, there are so many present in the family, it would just cause needless clutter to look at them today. There are a few straightforward discrete traits I'm not assessing either, mainly because things like the number of teeth poking out, baldness or whether or not the kid needs glasses are kinda... boring.
Step 2: Genetics 101
With all that our of the way, we can finally move on to the actual genetics, but even simplified as much as possible, there's still a fair bit of science involved, so I believe a quick tutorial is in order. (And considering how I'm condensing three years of genetics classes into a few paragraphs here, yes, it is short.)
First of all, you don't actually have "the gene for blue eyes" or "the gene for red hair" or whatever: you have genes for eye colour and hair colour, but what actually determines the colour are called alleles. Every gene (as far as we're concerned here) has multiple alleles - i.e. for the eye colour gene, there are alleles for blue eyes, green eyes, brown eyes, etc. On a more technical level, genes are sections of DNA, which are long winding coils of, essentially, coding, meaning alleles are equivalent to alternate chunks of coding. Makes sense, right? If the eye colour section of DNA has the code for blue eyes, and you get blue eyes; if the DNA codes for green eyes, you get green eyes, etc. So far so good? Because now it gets complicated...
DNA is grouped together into structures known as chromosomes, and everyone has two copies of every chromosome - one from your mother, one from your father. (On a side-note, don't confuse this with the fact that DNA is double-stranded: the two copies from your two parents are two completely separate pieces of DNA.) This means that you have two copies of every single gene, and this has a huge impact on what you end up looking like. If the allele is the same in both copies of the gene, then you're known as homozygous, and more importantly, you express that given trait, simple as that (i.e. two copies of the blue-eyed allele = blue eyes). However, if you're heterozygous and have different alleles, it's not so straightforward, and your genotype (what alleles you have) might not be the same as your phenotype (what you look like).
complete dominance | R | b |
---|---|---|
R | RR | Rb |
b | Rb | bb |
incomplete dominance | R | B |
R | RR | RB |
B | RB | BB |
codominance | R | B |
R | RR | RB |
B | RB | BB |
The chart on the left shows the basic results you get when you combine two different alleles. You see, not all alleles are created equal, resulting in one allele being dominant over the other allele. The most basic form is complete dominance, where if one allele is present, what it codes for is what gets expressed, regardless of what the other allele is. In the chart's top section, you can see how if the "R" allele is present, the colour that results is red, and you only see the blue phenotype if there are no "R"s, only two "b" alleles. (By the way, in this and all the other tables, the dominant gene, i.e. "R", is uppercase, while the recessive gene, i.e. "b", is lowercase.) In real life, eye colour is an example of this: if you have the allele for brown eyes, you will have brown eyes, end of story. Unfortunately, complete dominance isn't the end of our story, since you can also have incomplete dominance, where the one allele isn't overpowering enough to completely mask the second allele, resulting in an intermediate quality being expressed in heterozygotes. In the middle section of the chart, having an "R" allele and a "B" allele means you're not red or blue, but purple. Codominance works the same way, only instead of the two traits blending together into something new, you actually see both traits at work: in the chart, the "RB" heterozygotes are red and blue instead of purple, and in real life, this is why you get AB type blood as well as plain A and B types. For the sake of argument, we're only going to involve complete and incomplete dominance in this exercise, but it's still good to know the full story, n'est pas?
complete dominance | |||
---|---|---|---|
R | b | y | |
R | RR | Rb | Ry |
b | Rb | bb | by |
y | Ry | by | yy |
incomplete dominance | |||
R | B | Y | |
R | RR | RB | RY |
B | RB | BB | BY |
Y | RY | BY | YY |
Of course, most genes have more than two alleles, but for simplicity, we're only going to go up to three alleles in this article, and the chart on the right shows the basic results you get. Complete dominance is still nice and simple: if you have "R", you are red; if you have "b" but no "R", you're blue; if you have nothing but "y"s in your genotype, you're yellow. (Incidentally, since the English alphabet only has two cases, I can't differentiate between the intermediate "b" and completely recessive "y", but I'm hoping the fact that "y" dips below the line while "b" sticks up is enough to infer the hierarchy.) Incomplete dominance is still intuitive too: homozygotes express the primary colours, while heterozygotes blend their alleles' colours together into purple, green or orange phenotypes depending on the combination. However, just as alleles aren't equally dominant, how they react with other alleles also isn't consistent. For example, even though the aforementioned "A" and "B" blood type alleles are codominant to each other, they're both completely dominant over a third, "o" allele, meaning the only way you can have type O blood is if you're homozygous for "oo". But like codominance, this level of complexity isn't going to be involved in the Koopa analysis - I'm just bringing it up to give you a better idea of how complex genetics is. It's fun stuff. Nerdy, nerdy fun.
Step 3: Simplify some more!
Even with each trait only being controlled by one gene with three possible alleles and only complete or incomplete dominance, this still leaves us with a tonne of potential patterns for the crosses. Fortunately, we can whittle it down a bit more by stating that Bowser and the mother are both heterozygotes, since if either one were homozygous, they'd only be able to produce offspring with two phenotypes at most. In fact, if the parent was homozygous for a completely dominant allele, or if the second parent was also a homozygote, all the kids would look the same - and that's definitely not the case.
We can also assume the mother doesn't have any colours not seen in the Koopalings, and similarly, we can assume that all the phenotypes we see in the Koopalings are all the phenotypes we can see. I.e. if there's three eye colours, we don't assume there could be a fourth colour whose particular combination of alleles, while possible, didn't come up. Both of these assumptions cut down on excess fan speculation, and the added constraints makes it easier to zero in on the genetic pattern we're looking for.
Step 4: Solve the Puzzles
All this leaves us with a manageable amount of potential patterns to choose from (but for space, I won't listen them here), and we can finally start figuring out the genetics of the Koopa family.
Head and Old Shell Colours
Head colour phenotype | Bowser | ||
---|---|---|---|
? | ? | ||
??? | ? | 5 | 1 (2) |
? |
First we'll start with the simplest trait: head colour. And, if we assume Wendy's head is the same colour as her body because she's a female, rather than being gold because of a gold-coding genotype, the head colour phenotype pattern is the same as the phenotypes for the old shell designs - so we can kill two birds with one stone. Another thing we can do to simplify matters is ignore Morton's weird piebald light-and-dark colouration as a mutation - it's not a crucial step here, but it will be for some of the other traits, and it's best to be consistent throughout the analysis, so out he goes. Therefore, we only have two relevant phenotypes seen in the offspring, meaning we only have a couple potential genotype patterns to choose from, which are shown below.
Head colour genotypes | |||
---|---|---|---|
Complete dominance 1 | Bowser | ||
A | b | ||
??? | A | AA | Ab |
b | Ab | bb | |
Complete dominance 2 | Bowser | ||
A | b | ||
??? | b | Ab | bb |
c | Ac | bc |
Each individual 4x4 square is known as a Punnett square, and shows how the potential genotypes of Bowser and the unknown mother can combine to form genotypes that yield the phenotypes we see in the Koopalings. In this case, we only had to use simple complete dominance to explain how green-shelled Bowser could produce green- and pink-shelled Koopalings: the dominant "A" allele codes for green shells, the intermediate "b" allele codes for pink shells, and what "c" codes for is never seen because "c" is recessive (but like the "y" allele from the tutorial, there's no third case to distinguish "b" and "c", so bear with me). Since we don't know what the mother looks like, this gives us some flexibility, and in this case, she could be either green-shelled like Bowser, or pink. In this situation alone, she could even be homozygous for "b", as this would produce the same phenotypic pattern in the Koopalings as the "Complete dominance 2" pattern.
Now, once we have a couple options, we can go a step further and determine which pattern is better. As you'll soon see, this isn't always possible, but it is here, because of the numbers shown in the left-hand square. Five of the seven assessed Koopalings (about 70%) have green heads and former shell colours, whereas only one has a pink head and two had pink shells. Now, looking at the crosses on the right, we see that the first option produces green Koopalings with 3/4 allele combos while the second option has a 2:2 ratio. Seeing as 70% is a lot closer to 75% than 50%, it's more likely that the "Complete dominance 1" pattern is the right one, making the mother green-shelled. This is also advantageous in that we don't have to worry about what the "c" allele codes for, since only "A" and "b" are involved now.
New Shell Colours
Phenotypes | |||
---|---|---|---|
New shell colour | Bowser | ||
? | ? | ||
??? | ? | 2 | 2 |
? | 1 | 2 | |
Spike ring colour | Bowser | ||
? | ? | ||
??? | ? | 2 | 2 |
? | 1 | 2 |
After the redesign, the Koopalings' shells got a lot more colourful, with purple, blue and orange joining green and pink as the phenotypes. The rings at the bases of the original seven kids' shell spikes also became colourful, now coming in orange, yellow, pink, purple and blue, instead of plain brown like Bowser and Junior. However, as we've seen in the above Punnett squares, a cross of a single gene can only produce four distinct genotypes, and thus, four phenotypes. This means that simply ignoring Morton's weird shell isn't enough: we have to group a couple of the more similar colours to get the options down to four. As seen in the squares to the left, pink and purple get combined, as do the red and orange rings and the different shades of greens and blues that occur. Obviously one or more additional genes will need to determine whether, for example, the colour comes out purple or pink, but we're keeping this simple, and while a cross with two genes would produces 16 genotypes (more than enough to cover all the Koopalings' colours), it's a lot more overwhelming to the untrained eye, so we're just gonna stick to colour grouping today.
Spike ring colour genotypes | |||
---|---|---|---|
Incomplete dominance 1 | Bowser | ||
A | b | ||
??? | A | AA | Ab |
c | Ac | bc | |
Incomplete dominance 2 | Bowser | ||
A | b | ||
??? | A | AA | Ab |
c | Ac | bc | |
Incomplete dominance 3 | Bowser | ||
A | b | ||
??? | A | AA | Ab |
c | Ac | bc |
New shell colour genotypes | |||
---|---|---|---|
Incomplete dominance 1 | Bowser | ||
A | b | ||
??? | A | AA | Ab |
c | Ac | bc | |
Incomplete dominance 2 | Bowser | ||
A | b | ||
??? | A | AA | Ab |
c | Ac | bc | |
Incomplete dominance 3 | Bowser | ||
A | b | ||
??? | A | AA | Ab |
c | Ac | bc |
Our four combined phenotypes can be explained by six different genotype patterns, but unlike with the old shell / head colour situation, all the crosses result in the same phenotypic pattern in the offspring, so I only bothered showing one pattern. However, while what the alleles coded for were fixed in the old shell case, for both the new shell and spike rings, it's the combinations that matter, not the alleles themselves, and as long as Bowser's two pairs of alleles make him green-shelled and brown-ringed, all the other pairs could code for any of the other colours. Conversely, as long as the mother isn't green-shelled and brown/orange-ringed, she could have any combination of any of the colours of shell or rings seen in the Koopalings, which is what's being shown by the inclusion of three Punnett squares for both the New Shell and Spike Ring patterns. While the genotypes remain the same for both Bowser and the mother in all three squares, the different combinations have been assigned different colours, showing the three possible colours the mother could have for each trait (then just mix n' match to get your lady's looks).
And that's not even the half of it: for every square, "AA" and "bc" can actually have their colours swapped, since neither combination is seen in the parental genotypes, but since that doesn't add anything to our analysis, I left those squares out. This is also why I left out the other five genotype patterns: they're all exactly the same as the one shown, but with different combinations of alleles for the parents (i.e. Bowser is "bc" instead of "Ab", or he and the mother are swapped with him being "Ac" and her being "Ab", etc.).
Now, one disadvantage of combinations being the determinants for the colours is that the alleles themselves can't be assigned colours, otherwise you'd get situations like "blue + yellow = pink", which doesn't make sense. However, genes don't actually code for colours, they code for proteins, which then go on to react with other proteins to make the colours. So, while we can't use the colour wheel to figure this one out, the idea that Bowser can indeed produce a veritable rainbow of offspring is still scientifically sound.
Eye Colour
Eye colour phenotype | Bowser | ||
---|---|---|---|
? | ? | ||
??? | ? | 1 | 2 |
? | 3 |
Much less varied are the Koopalings' eye colours: three are clearly blue-eyed, and if you zoom in on the recent artwork, you'll see grey rings in the irises of all the formerly dot-eyed Koopalings (except the pure-black-eyed Lemmy, but we're ignoring him as a mutant; we also have to disregard Roy since we simply can't see his eyes at all). Assuming Junior's resemblance to Bowser extends to his eyes, and we just can't see the red irises because he's a baby, that gives is a total of three phenotypes, shown in the square to the left.
Eye colour genotypes | |||
---|---|---|---|
Incomplete dominance | Bowser | ||
A | B | ||
??? | A | AA | AB |
B | AB | BB | |
Complete dominance | Bowser | ||
b | c | ||
??? | A | Ab | Ac |
c | bc | cc |
Three phenotypes can be explained by six possible crosses, but these fall into the two overall patterns shown on the right. The first pattern is incomplete dominance when the parents have the same genotype, which works for every pair of alleles (i.e. the parents could be "BC" or "AC" as well as the "AB" genotypes shown). The second pattern is complete dominance, which only works if one parent is "bc" and the other is "Ac" (in the genotype shown, Bowser is the "bc" one). For complete dominance, one allele can code for red, one for blue and one for grey, but for incomplete dominance, like with the new shell colours, the combinations, not the alleles, are what determines the eye colour. Either way, while Bowser's allele combo always has to correspond to red, which genotypes result in blue and which ones result in grey can be swapped, meaning the mother could have either colour if the dominance is complete (whereas in the incomplete dominance pattern means she has to have red eyes like Bowser).
Unlike the new shell colour crosses, the eye colour patterns here do not yield four phenotypes that occur with equal likelihood: instead, one eye colour has twice the odds of occurring than the other two. The differences in the amount of each phenotype that occur in the Koopalings isn't as extreme as in the old shell / head colour situation, but if one phenotype has to be more likely, it might as well be blue - the one that is seen a little more often. With this in mind, we can say complete dominance and a blue-eyed mother is the more likely situation, which works out well because complete dominance is the simplest pattern, and it allows for the alleles themselves to be the colour determinants, simplifying things even further.
Hair Colour
Phenotypes | ||||
---|---|---|---|---|
Old hair colour |
Bowser | |||
? | ? | |||
??? | ? | 1 | 2 | |
? |
|
|||
New hair colour | Bowser | |||
? | ? | |||
??? | ? | 1 | 2 | |
? |
|
1 |
Like the shell colours, the Koopalings' hair colours changed a bit after their redesign: including Junior, the original colours were blue, red and rainbow hair, but the new designs changed Iggy's hair to green and made Larry's blue a bit fancier (but it's still blue, so no problem). It also changed Wendy's eyebrows from colourless lines to blonde lines, but this bumps the number of phenotypes up past four, even if we ignore Morton's still-colourless black lines. Therefore, like the shells, we have to lump Wendy in with another colour; where she goes doesn't really matter, but given how Lemmy's ponytail is yellow, it could be that excess hair in a rainbow genotype is yellow, including Wendy's blonde, so I just grouped her there.
Old hair genotype | |||||||||
---|---|---|---|---|---|---|---|---|---|
Complete dominance | Bowser | ||||||||
b | c | ||||||||
|
A |
|
| ||||||
c | bc | cc |
New hair genotype | |||||
---|---|---|---|---|---|
Incomplete dominance | Bowser | ||||
A | C | ||||
??? | B |
|
BC | ||
C | AC | CC |
Since it has three phenotypes showing up in the offspring, the old hair colour is the same situation as eye colour, so for simplicity, I only showed our trusty complete dominance pattern. In this genotypic pattern, "A" is rainbow, "b" is red and "c" is blue, but the "A" and "c" values can be swapped to make the mother's hair blue instead. In an incomplete dominance pattern, the mother would have red hair like Bowser, just like the shared red eyes, but there's no need to go over the same thing twice, so I didn't show that cross here. As for which pattern is more likely, that would be complete dominance (since incomplete dominance would favour red eyes in the offspring, but that phenotype comes up the least), but since blue and rainbow hair occur the same number of times, which one is also the mother's hair colour is anyone's guess: both would work equally well.
The new hair, on the other hand, has four options, making it the same situation as the new shell colour. The mother, therefore, could have blue, rainbow or green hair, but seeing as we've been down this road before, I only bothered showing a cross that makes her blue-haired. However, while I'm admittedly biased towards blue, there's a good reason for using this as the example, because if we make "C" recessive instead of incompletely dominant like the others, that means that while the "A" allele codes for red and the "B" allele codes for blue, when put together, you get rainbow hair (which is actually the exact same pattern as the blood type stuff in the tutorial). Granted, rainbows have other colours besides red and blue, but like I said before, proteins work in mysterious ways sometimes, and besides, it's better than simply saying the combination's what matters, because this way, we can attribute the colours to the alleles, and as always, the simplest solution to the puzzle is the best one.
Step 5: Sit Back and Relax
So there you have it, a complete rundown of all the colours seen in the would-be Koopa family, and the genetic patterns that could be responsible for them. Genetic analysis is tricky stuff, even simplified to the most basic level like I've done here, but as you can see, you can do neat stuff with it if you have the time and patience to work through it all. To me, showing that Bowser and the seven Koopalings could have been related all along was always a worthy task, and I hope you guys found it interesting as well. But even if the technical details are a little fuzzy, just remember, it is possible for a plain old Koopa like Bowser to have produced a crop of kids as colourful as the Koopalings (including Junior). So whether or not you accept Nintendo's "current" story, and regardless of if you even want Bowser's family to include the seven original Koopalings, keep one thing in mind: they could be related, and whether they are or not... Well, that all depends on you, and what you make of this twisty little aspect of the Mario mythos.